Research
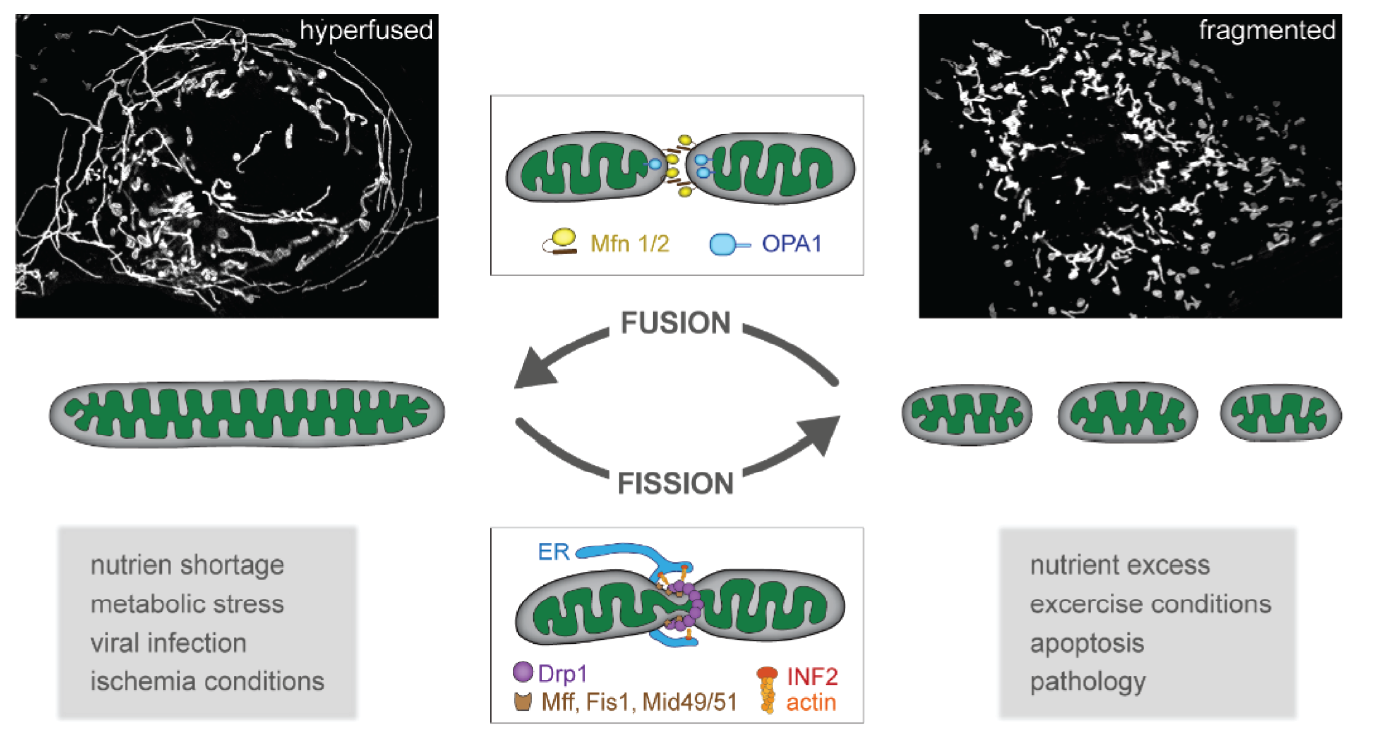
Mitochondrial fission and fusion are central processes in adapting mitochondrial function and regulating cellular energy homeostasis. The inability of mitochondria to adapt their morphology leads to bioenergetic defects and an accumulation of damaged mitochondria. However, the precise mechanism how altered mitochondrial structure and imbalances in mitochondrial dynamics lead to bioenergetic dysfunctions is poorly understood.
Using a powerful combination of quantitative super-resolution imaging of mitochondrial biosensors and proteomic approaches, we examine the link between mitochondrial ultrastructure and metabolic function. Our preliminary data suggest, that mitochondria are highly dynamic in proliferating cells, which mainly rely on glycoslysis. Upon a metabolic switch from glycolysis to oxidative phosphorilation, for example during cell differentation, mitochondria lose plasticity and become more static.
Understanding the link between mitochondrial dynamics, morphology and metabolic function will also enhance our understanding of how morphological aberrations of mitochondria lead to bioenergetic defects in pathology and might point towards novel strategies to re-balance mitochondrial dynamics and metabolic function in disease states.
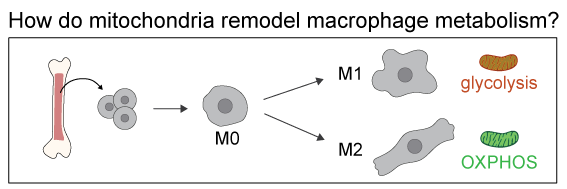
As a model system, we use bone-marrow derived murin macrophages polarized into pro-inflammatory and anti-inflammatory macrophages
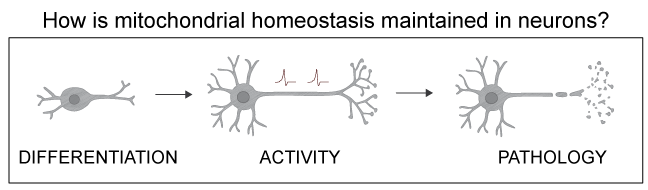
In neurons, mitochondria face particular challenges. The human brain only accounts for 2% of the body mass, but it comsumes over 20% of the body's oxygen to meet its high metabolic demand. A single neuron in the substantia nigra contains over two million mitochondria. At the same time, neurons are not renewed over a humans' lifetime, maening that oxidative damage and mtDNA mutations excaping quality control accumulate over decades. In addition neurons have a complex architecture, where places of energy demand are far away from the cell body, posing challenges for distributing and maintaining a healthy mitochondria pool.
Hence it is not surprising, that disbalances in mitochondrial fission and fusion primarily manifest in neurological diseases. However, studies examing mitochondrial dynamics in neurons are extremly rare. Several neurodegenerative disease, such as Alzheimer's and Parkinson's disease, but also aged neurons are characterized by a fragmented mitochondrial network. However, if this fragmentation is cause or consequence of disease is still obscure.
To gain a deeper understanding of how mitochondrial dynamics are regulated in neurons and how disbalances in these processes result in pathology, we are using live-cell super-resolution imaging of murine primary neurons and human iPSC derived neurons.
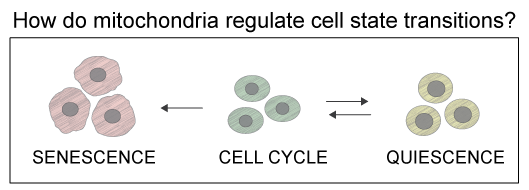
Transitions into distinct cellular states, such as quiescence, terminal differentiation or senescence, require fundamental metabolic reprogramming and metabolic remodeling. Mitochondrial dynamics, through fission and fusion, facilitate structural and functional adjustments of cellular metabolism and are associated with cell state changes. Accumulating evidence implies that mitochondria activley drive cell state transitions and are causally linked to cellular fate. Nevertheless, most studies on mitochondria dynamics are performed in cycling cells but little is known on how mitochondria alter their behavior when cells exit the cell cycle and become quiescent, senescent or differentiate.
The aim of this project is to reveal how mitochondria are remodeled to satisfy the demand of different cell states and to what degree they actively drive cell fate transitions. As a model system, we use diploid, non-transformed hTERT-immortalized retinal pigment epithelial cells (RPE1) and induced pluripotent stem cells (iPSCs) in which we induce cell state transitions.
We have established a number of super-resolution based methods to investigate mitochondrial dynamics, ultrastructure and metabolic function at the single organelle level. For this we use live-cell structured illumination microscpy (SIM) and instant SIM (iSIM) as well as live-cell Stimulated Emission Depletion (STED) microscopy in different model systems, including iPCS derived neurons, stem cells and bone marrow derived macrophages. For quantitative analysis, we are developing AI-based automated image analysis pipelines to segment mitochondria and automatically detect fission and fusion events.
To identify regulators of mitochondrial structure and function, we are currently developing a live-cell super-resolution imaging-based screen to test the effect of small compounds and kinase inhibitors with high temporal and structural resolution.
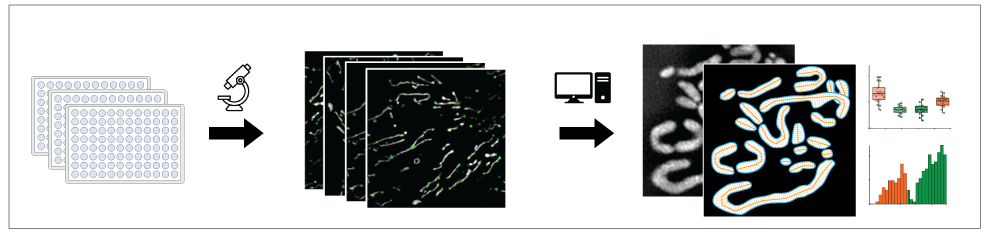